Arsenic
- Elements
Doublet Separations
- As 3d: 0.7 eV
- As 3p: 4.8 eV
- As 2p: 35.8 eV
The Energies Listed are Binding Energies!
As 3d: 41 eV
As 3p: 141 eV
As 3s 204 eV
As 4d: 3 eV
The Energies Listed are Binding Energies!
Overlaps for As 3d (primary emission)
- V 3p (38 eV)
- Hf 4f (38 eV)
- Ce 5s (38 eV)
- Pr 5s (38 eV)
- Sr 4s (38 eV)
- Pm 5s (38 eV)
- Nd 5s (38 eV)
- Sm 5s (39 eV)
- At 5d (39 eV)
- Te 4d (40 eV)
- At 5d (40 eV)
- Th 6p (43 eV)
- Cr 3p (43 eV)
- Ru 4p (43 eV)
- Ca 3s (44 eV)
Overlaps for As 3p
- P 2p (135 eV)
- Sn 4s (137 eV)
- Zn 3s (137 eV)
- Tl 5s (137 eV)
- Pb 4f (138 eV)
- Fr 5p (140 eV)
- Gd 4p (141 eV)
Energies listed are Kinetic Energies!
As LMM: ~ 1225 eV
The Energies Listed are Binding Energies!
Species | Binding energy / eV | Charge Ref | Ref |
As Metal | 41.5 | 2,3 | |
GaAs | 40.9 | 3 | |
As2O3 | 44.9 | C 1s (284.8 eV) | 4 |
As2O5 | 46.2 | C 1s (284.8 eV) | 4 |
NiAs | 41.1 | C 1s (284.8 eV) | 5 |
Species | Binding energy / eV | Charge Ref | Ref |
As Metal | 41.5 | 2,3 | |
GaAs | 40.9 | 3 | |
As2O3 | 44.9 | C 1s (284.8 eV) | 4 |
As2O5 | 46.2 | C 1s (284.8 eV) | 4 |
Arsenic is primarily analysed using the As(3d) region, which can overlap with the Ta(5p1/2) signal. Generally observed as an oxide or in GaAs, the As(3d) region has a spin-orbit splitting of 0.7 eV, with an intensity ratio of 0.7, as opposed to the expected 0.67.
Recording the As(2p3/2) region is useful also and demonstrates oxide thickness as a function of kinetic energy (see below)
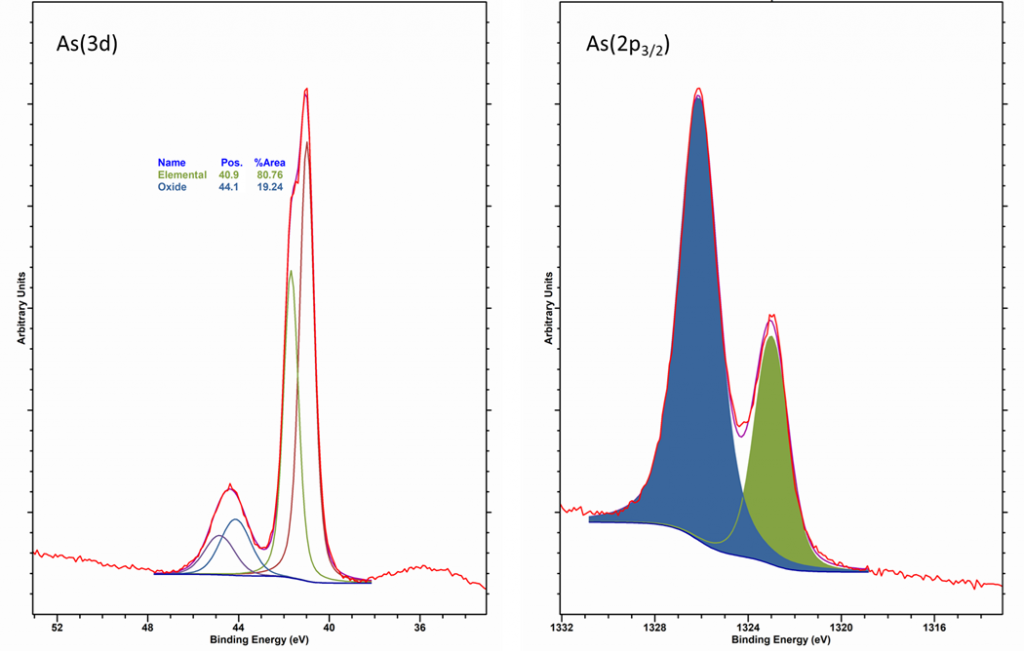
It has been reported that As2O3 sublimes in vacuum [1], whereas As5+ may under go some degredation by XPS analysis to yield As3+, so rapid analysis and/or summation of multi-point analysis may be required.
The As LMM Auger peaks when recorded with a Al source encompass a wide energy range (500 – 250 eV) and therefore overlap with many other photoemission lines, so care should be taken in analysis. This can be seen in the spectra below of As2O5.
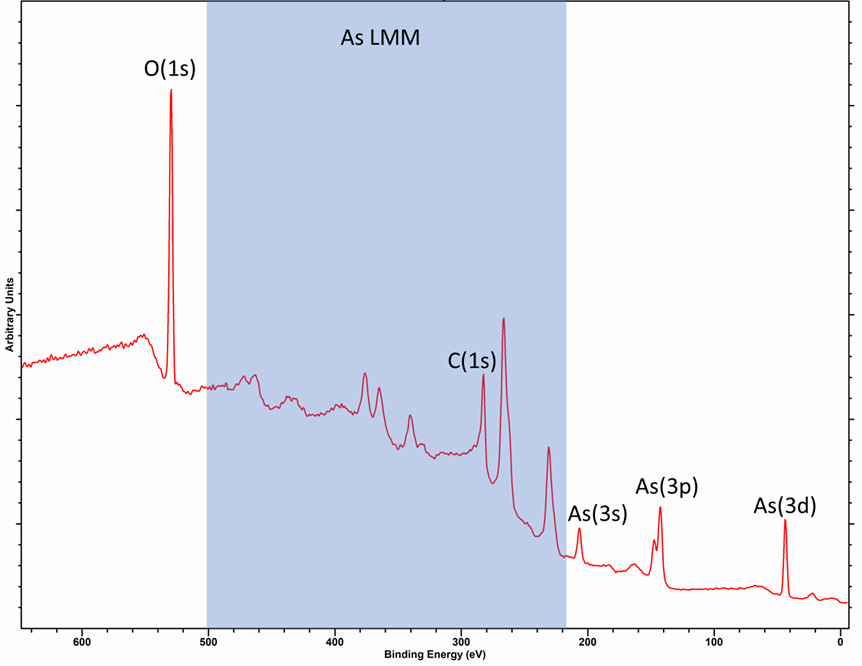
Due to the difficulties mentioned above, it is therefore common to use the modified auger parameter (α‘) to assign chemistry, values for which may be found in table 1. To find auger parameters of many more compounds, see reference 5 from Mark Biesinger.
Species | Modified auger parameter / eV | Ref |
Cu | 1851.2 | 2 |
Cu2O | 1849.4 | 3 |
CuO | 1851.5 | 4 |
Cu(OH)2 | 1853.1 | 5 |
CuCl | 1847.8 | 5 |
CuCl2 | 1850.2 | 5 |
CuSO4 | 1851.4 | 5 |
The modified auger paramater may also provide insight into specific nanoparticle chemistry via estimation of the relaxation energy (r).(6) This may be defined as half the change in the modified auger parameter compared to bulk Cu (equation 1).(7)
r = 0.5 * (|1851.2 – α‘) Equation 1
Copper nanoparticles may evidence increased relaxation energies when an decreased number of copper atoms are screening the core-hole (i.e. smaller nanoparticles)(1) or due to a decrease in the polarizability of the support.(8)
Copper metal does not exhibit a high degree of asymmetry, due to it’s largely filled d-band. A lineshape of LA(1.05, 110) can be used to give a good fit for Cu metal.
Figure 2: Cu metal fit with LA(1.05, 110) lineshape
When fitting copper doublets – do not constrain the FWHM of the doublets to be equal – due to the aforementioned Coster-Kronig broadening.
References
1. Arsenic entry at Thermo Scientific XPS Periodic Table
2. Arsenic entry at www.xpsfitting.com
3. Measured at HarwellXPS on Thermo Scientific K-Alpha+
4. Measured at HarwellXPS on Kratos Axis Ultra DLD system
5. H.W. Nesbitt and M. Reinke, American Mineralogist, 84 (1999) 629. Read online