- Elements
Carbon
Carbon analysis by XPS is typically recorded for every experiment, due to the common presence of adventitious carbon contaminant layers. Analysis is performed on the C 1s region, which may overlap with K 2p (only higher BE species) and Ru 3d.
Since carbon peaks are of S-orbitals analysis is often relatively trivial, with large energy separations between carbons of differing functional groups (figure 1)
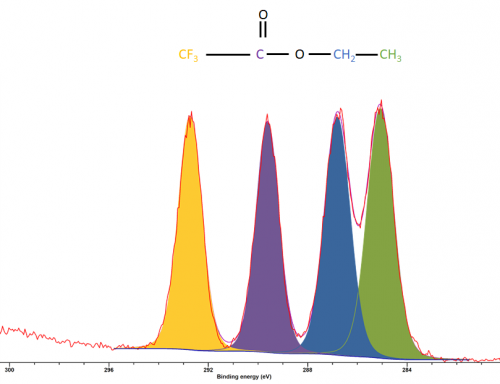
Carbon binding energies follow the typical trend of an increasing binding energy with a increase of the electronegativity of the nearest neighbouring atoms (figure 2).

Binding energies and references may be found in table form below:
Species | Binding energy / eV | Charge Ref. | Ref. |
sp3 C | 285 | C 1s = 285 eV | 1 |
sp2 C (graphitic/graphene) | 283.6 | Au 4f / 83.95 eV | 2 |
C=C | 284.2 | C 1s = 285 eV | 3 |
C – C(=O)R | 285.5 ± 0.3 | C 1s = 285 eV | 1 |
C-OH | 286.5 ± 0.2 | C 1s = 285 eV | 1 |
C-O-C | 286.5 ± 0.1 | C 1s = 285 eV | 1 |
C-N | 285.9 ± 0.1 | C 1s = 285 eV | 3 |
Epoxide | 287 | C 1s = 285 eV | 1 |
C=O | 287.8 ± 0.2 | C 1s = 285 eV | 1 |
C – C(O)=O | 286.8 ± 0.2 | C 1s = 285 eV | 1 |
O-C=O | 289 ± 0.2 | C 1s = 285 eV | 1 |
C-F2 | 292.6 | C 1s / 285 eV | 4 |
Metal-C | ~282 | C 1s / 284.6 eV | 5 |
Si-C | 283 | C 1s / 284.6 eV | 6 |
Graphene, graphite, graphene oxide and reduced graphene oxide
Novel 2D carbon materials are incredibly powerful technologies for a number of applications and as such require chemical analysis, often by XPS.
The choice of energy calibration for pure carbon samples is not an easy one, though peak shape is an early indicator of graphitic carbon vs other forms since graphitic carbon possesses an asymmetric peak shape with a lower FWHM than that of hydrocarbons or similar.
Aligning the major carbon peak to a typical binding energy once you have determined the character allows facile addition of additional components.
Further evidence for sp3 vs sp2 graphitic character may be obtained from the C KLL auger, specifically the differentiated spectra. Following differentiation, evaluation of the energy separation between the minima and maxima permits determination of a so-called ‘d-parameter’ which may be used to assess the carbon local environment (figure 3).
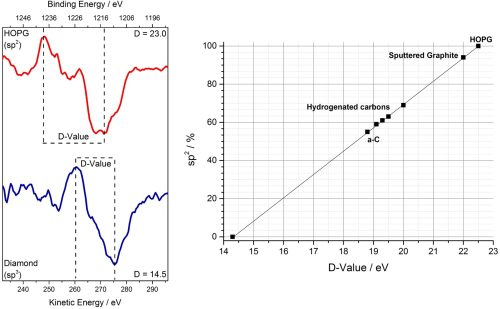
Graphitic carbon nitrides
Graphitic carbon nitrides (g-C3N4) is a unique family of materials commonly found in photocatalytic applications. The deconvolution of this structure is similar in many ways to pure graphitic carbon, but with some specific additional peaks. It may be difficult to energy calibrate such spectra to the lowest binding energy peak, since the majority of the spectra will be dominated by C-N-C peaks, so calibrating to this peak at 288.1 eV (figure 4).(7)
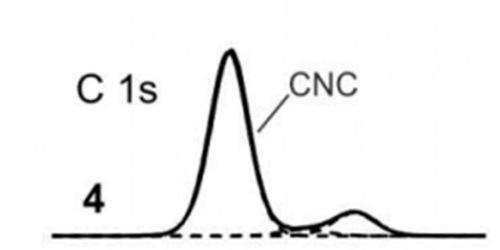
Polymers
Historically, XPS has found great use in the polymer community and as such a large database of polymer XPS can be found in the works of Beamson and Briggs especially.(1)
Inorganics (carbonates)
Carbonates are found at high binding energies (~289.5 ± 0.6 eV), with the exception being that of silver carbonate which may be found ~288.5 eV.
References
- Briggs, D. and G. Beamson (1992). “Primary and secondary oxygen-induced C1s binding energy shifts in x-ray photoelectron spectroscopy of polymers.” Analytical Chemistry 64(15): 1729-1736. Read it online here.
- Geng, D., et al. (2011). “Nitrogen doping effects on the structure of graphene.” Applied Surface Science 257(21): 9193-9198. Read it online here.
- Ederer, J., et al. (2017). “Determination of amino groups on functionalized graphene oxide for polyurethane nanomaterials: XPS quantitation vs. functional speciation.” RSC advances 7(21): 12464-12473. Read it online here.
- Santerre, F., et al. (1999). “Properties of TiC thin films grown by pulsed laser deposition.” Applied Surface Science 148(1-2): 24-33. Read it online here.
- Shimoda, K., et al. (2007). “Influence of surface structure of SiC nano-sized powder analyzed by X-ray photoelectron spectroscopy on basic powder characteristics.” Applied Surface Science 253(24): 9450-9456. Read it online here.
- Beamson, G., et al. (1996). “Characterization of PTFE on silicon wafer tribological transfer films by XPS, imaging XPS and AFM.” Surface and Interface Analysis: An International Journal devoted to the development and application of techniques for the analysis of surfaces, interfaces and thin films 24(3): 204-210. Read it online here.
- Qiao, S., et al. (2016). “Pore confinement effects and stabilization of carbon nitride oligomers in macroporous silica for photocatalytic hydrogen production.” Carbon 106: 320-329. Read it online here.
Copper
- Elements
Doublet Separations
- Cu 2p: 19.8 eV
- Cu 3p: 2.4 eV
The Energies Listed are Binding Energies!
Cu 2p: 933 eV
Cu 2s: 1096 eV
Cu 3s: 120 eV
Cu 3p: 74 eV
The Energies Listed are Binding Energies!
Overlaps for Cu 2p (primary emission)
- Cs MNN (Al kα X-rays) (927 eV)
- I 3p (931 eV)
- Pr 3d 5/2 (932 eV)
- Bi 4s (939 eV)
- Sb 3s (944 eV)
- Mnn LMM (Al kα X-rays) (947 eV)
- Pr 3d 3/2 (953 eV)
Overlaps for Cu 3s
- Pr 4d (114 eV)
- Nd 4d (118 eV)
- Al 2s (119 eV)
- Pm 4d (121 eV)
- In 4s (122 eV)
- Ge 3p (122 eV)
- I 4p (123 eV)
Energies listed are Kinetic Energies!
Cu LMM: ~ 569.6 eV
The Energies Listed are Binding Energies!
Species | Binding energy / eV | Charge Ref | Ref |
Satellite peaks are often observed in the Cu 2p spectra due to the presence of unpaired electrons in the 3d orbitals. Copper has a partially filled 3d orbital, which makes it susceptible to multiplet splitting. The presence of unpaired electrons in the 3d orbital leads to complex interactions within the electron cloud surrounding the copper atom.
Cu metal and Cu2O have a closed 3d shell, and hence exhibit little or no multiplet splitting/final-state effects. CuO on the other hand, has an open d shell (3d9) and is prone to these.
CuO exhibits a significant satellite around 9 eV above the major photoemission core line, characteristic of 3d9 states. The structure seen in the satellite line is
due to the multiplet splitting in the 2p53d9 final state. Explanations of the satellite in CuO (and the lack of it in Cu2O) have been developed using a cluster model in which the d-shell of CuO (being unfilled) accept charge transfer from neighbouring oxygen bands to screen the core-hole created via photoemission (creating a 3d10L) configuration (note that L. refers to a core-hole in the ligand) This results in the well defined satellite structure seen in CuO. The d-shell of Cu2O is full so screening via a charge transfer into the d states is not possible. The screening of the core hole must then be accomplished by states involving the broad sp conduction band and will, therefore, not yield sharp satellite structures.(9)
When analysing copper it is imperative that you record the Cu LMM auger as well as the Cu 2p region. This is because the difference between Cu0 and CuI XPS spectra is very slight, and correct speciation identification may prove impossible without the auger region to assist (Figure 1).
Figure 1: Cu, Cu2O and CuO 2p and LMM auger XPS(1)
Detailed guides on fitting carbons may be found below:
- Comments on the XPS analysis of carbon materials – David J. Morgan
- Practical guides for x-ray photoelectron spectroscopy (XPS): Interpreting the carbon 1s spectrum – Thomas R. Gengenbach, George H. Major, Matthew R. Linford, Christopher D. Easton
- Accessing the robustness of adventitious carbon for charge referencing (correction) purposes in XPS analysis: Insights from a multi-user facility data review – Mark C Biesinger
References
- Islam, M. J., et al. (2020). “The effect of metal precursor on copper phase dispersion and nanoparticle formation for the catalytic transformations of furfural.” Applied Catalysis B: Environmental: 119062. Read it online here.
- Miller, A. and G. Simmons (1993). “Copper by XPS.” Surface Science Spectra 2(1): 55-60. Read it online here.
- Vasquez, R. (1998). “Cu2O by XPS.” Surface Science Spectra 5(4): 257-261. Read it online here.
- Vasquez, R. (1998). “CuO by XPS.” Surface Science Spectra 5(4): 262-266. Read it online here.
- Biesinger, M. C. (2017). “Advanced analysis of copper X‐ray photoelectron spectra.” Surface and interface analysis 49(13): 1325-1334. Read it online here.
- Thøgersen, A., et al. (2008). “An experimental study of charge distribution in crystalline and amorphous Si nanoclusters in thin silica films.” Journal of Applied Physics 103(2): 024308. Read it online here.
- Moretti, G. (1998). “Auger parameter and Wagner plot in the characterization of chemical states by X-ray photoelectron spectroscopy: a review.” Journal of Electron Spectroscopy and Related Phenomena 95(2-3): 95-144. Read it online here.
- Batista, J., et al. (2001). “On the structural characteristics of γ-alumina-supported Pd–Cu bimetallic catalysts.” Applied Catalysis A: General 217(1-2): 55-68. Read it online here.
- Ghijsen, Jacques, et al. “Electronic structure of Cu 2 O and CuO.” Physical Review B 38.16 (1988): 11322. Read it online here.