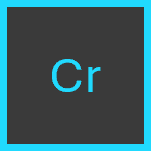
Chromium
Doublet Separations
- Cr 2p: 9.3 eV
The Energies Listed are Binding Energies!
- Cr 2s: 695 eV
- Cr 2p: 575 eV
- Cr 3s: 74 eV
- Cr 3p: 43 eV
- Cr 3d: 2 eV
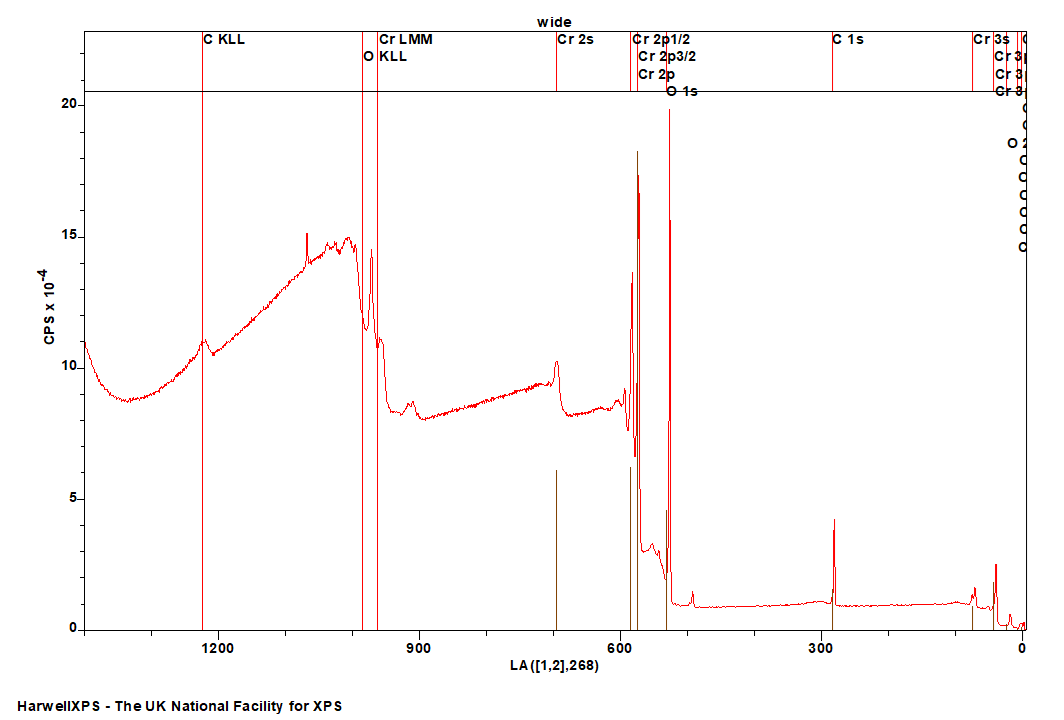
The Energies Listed are Binding Energies!
Cr is primarily analysed via the 2p orbital
- Cu LMM (Al source) (566 eV)
- Ta 4s (566 eV)
- Rn 4d (567 eV)
- Ag 3p (571 eV)
- Hg 4p (571 eV)
- Te 3d (572 eV)
- Fr 4d (577 eV)
- Ir 4p (577 eV)
- Ru 3s (575 eV)
- W 4s (595 eV)
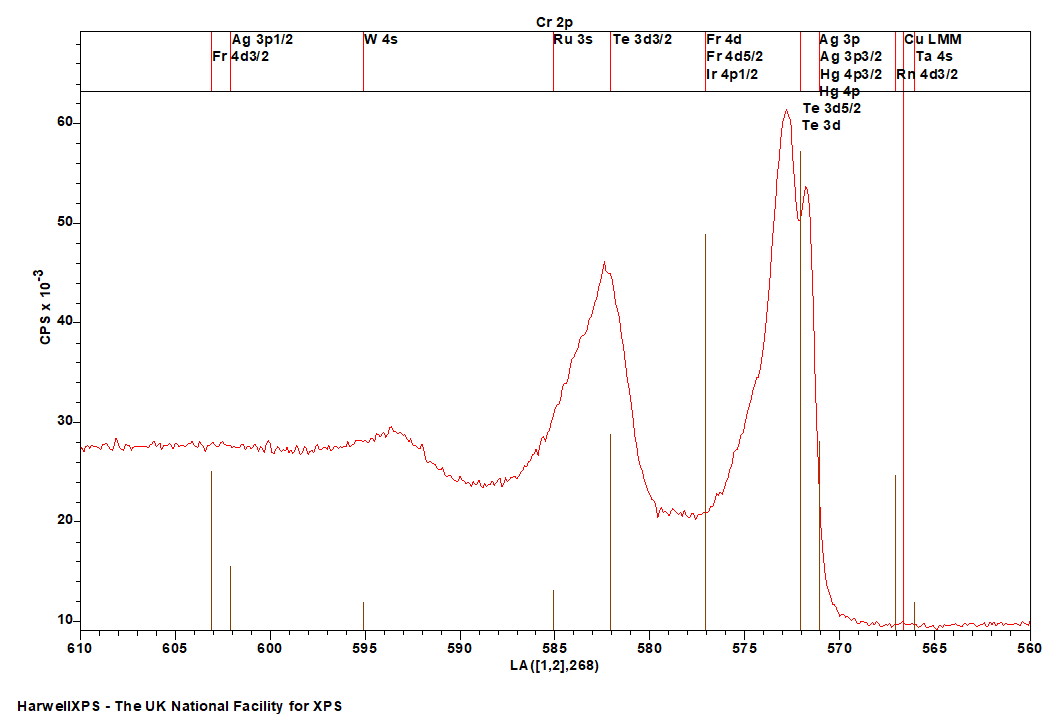
Energies listed are Kinetic Energies!
Note – Cr LMM may overlap with O KLL
Cr LMM: ~ 525 eV
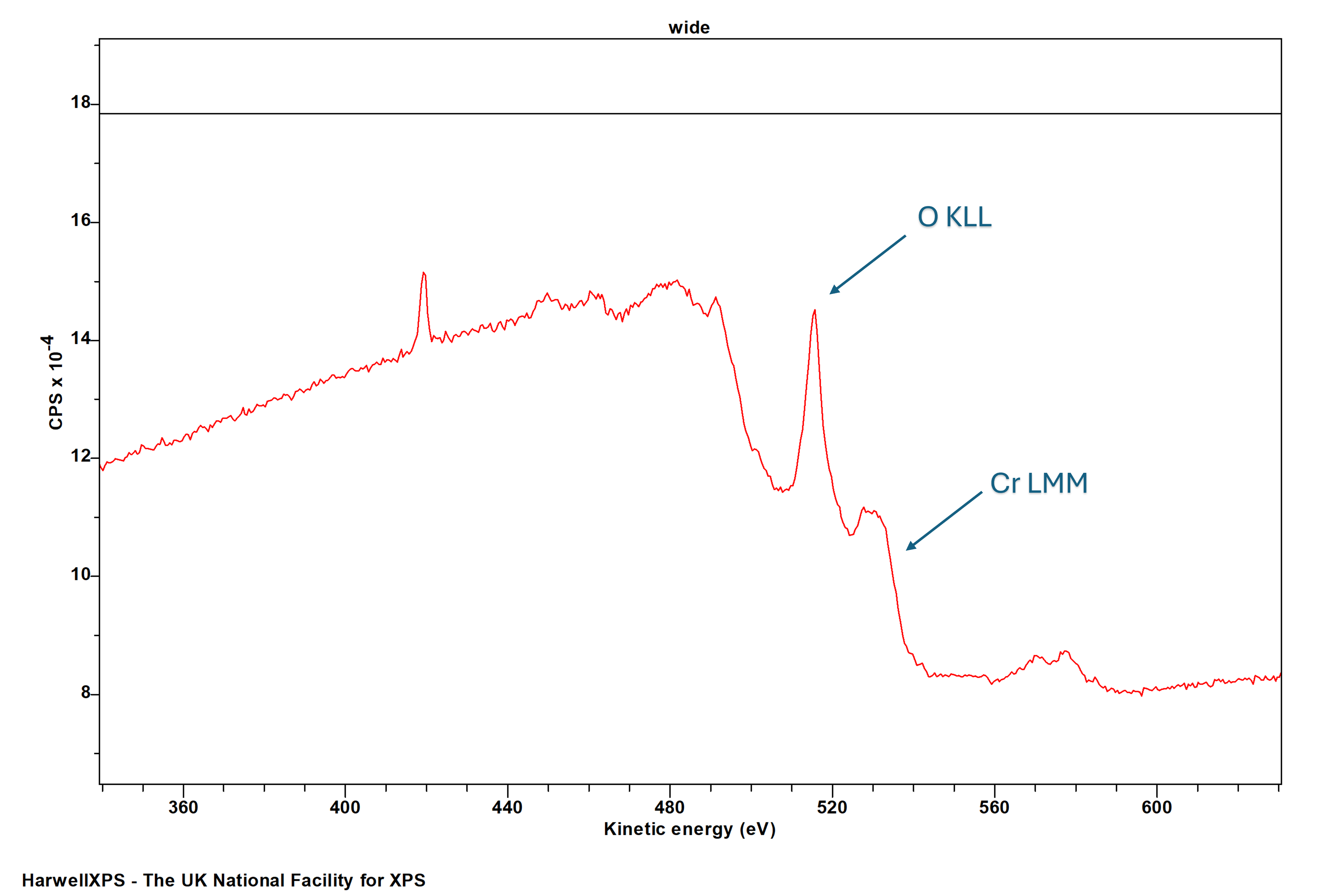
Chromium in particular oxidation states (most notably Cr(III) compounds, due to unpaired electrons) exhibits multiplet splitting. The 2p region exhibits broadening and asymmetry in a number of oxides, while the 3s region splits into two peaks in a manner indistinguishable when above or below the Curie temperature,[2] indicating that the ferromagnetic spin ordering does not influence this splitting. Cr(VI) is diamagnetic and therefore does not exhibit multiplet splitting. CrO2 is sometimes considered as a single pair of asymmetric peaks, due to being a conductive oxide[3] – however given the breadth of the peaks, and the paramagnetic [Ar]3d2 configuration of Cr(IV) it is considered possible to be due to multiplet splitting rather than a asymmetric shake-up processes.[4]
Chromium (VI) is known to photoreduce to tetra- and trivalent species under X-ray illumination.[5] This photoreduction can be greatly accelerated by certain charge neutralisation techniques – such as a dual neutraliser, and as such, care should be taken in selecting a neutralisation mode to minimise rapid sample damage.[6]
Fitting of Cr(III), much like any species with significant multiplet structure, can be a time-consuming task. Models developed from first-principles and standards can go a long way to help,[7] and recording your own data standards can help too.
Not available
Not available
- Salvi, Anna M., et al. “Peak fitting of the chromium 2p XPS spectrum.” Applied surface science 90.3 (1995): 333-341. Read it online here.
- Ikemoto, Isao, et al. “X-ray photoelectron spectroscopic studies of CrO2 and some related chromium compounds.” Journal of Solid State Chemistry 17.4 (1976): 425-430. Read it online here.
- Bullen, Heather A., and Simon J. Garrett. “CrO2 by XPS: comparison of CrO2 powder to CrO2 films on TiO2 (110) single crystal surfaces.” Surface Science Spectra 8.3 (2001): 225-233. Read it online here.
- Biesinger, Mark C., et al. “Resolving surface chemical states in XPS analysis of first row transition metals, oxides and hydroxides: Cr, Mn, Fe, Co and Ni.” Applied Surface Science 257.7 (2011): 2717-2730. Read it online here.
- Halada, Gary P., and Clive R. Clayton. “Photoreduction of hexavalent chromium during X‐ray photoelectron spectroscopy analysis of electrochemical and thermal films.” Journal of the Electrochemical Society 138.10 (1991): 2921. Read it online here.
- Edwards, Lee, Paul Mack, and David J. Morgan. “Recent advances in dual mode charge compensation for XPS analysis.” Surface and Interface Analysis 51.9 (2019): 925-933. Read it online here.
- Biesinger, M. C., et al. “X‐ray photoelectron spectroscopy studies of chromium compounds.” Surface and interface analysis: an international journal devoted to the development and application of techniques for the analysis of surfaces, interfaces and thin films 36.12 (2004): 1550-1563. Read it online here.